These are the words of Silvestro Micera, head of the bioengineering section of a highly advanced project at the Swiss Federal Institute of Technology in Lausanne, which has allowed three paraplegics to begin to walk again.
Images of 30-year-old paraplegic Michel Roccati walking again, albeit with support, after cutting-edge surgery in Lausanne, went around the world.
Another two paralysed people (out of the 27 patients who initially signed up for the trial) obtained the same exceptional result. This was thanks to a team led by Grégoire Courtine of the Swiss Federal Institute of Technology in Lausanne (EPFL), with the support of dozens of doctors, bioengineers and researchers from various countries.
The importance of their work, described in the scientific journal Nature Medicine, goes much further than its success in the lives of these initial volunteers. It concretely demonstrates what it means to carry out highly complex studies with determination for years and shows how much medicine is integrated with technology and STEM sciences (science, technology, engineering and mathematics), as well as the synergies that arise from this – by no means cold – fusion.
This result comes after more than a decade of experimentation, first on rats, then on primates and, finally, on humans. “What has changed and what gave the decisive impulse, was considering the system that translates a new stimulus into a movement in all its extraordinary complexity, and not focussing only on fundamental elements, as in the past,” explains Silvestro Micera of the Sant’Anna School of Advanced Studies in Pisa, head of the bioengineering part of the project and speaker at the IBSA Foundation’s 2016 forum entitled "New technologies to treat neurodisorders: neuroprosthetics”.
When there is a spinal injury, the signal from the brain cannot reach the nerve cells (and their extremities, called dorsal roots) that control muscle movement. Until now, attempts were made to pass an electrical impulse through the fibres below the lesion, using readapted prostheses originally intended to stop the transmission of pain stimuli. However, the results were not the best, due to the features of these prostheses (they were too short, made of silicone, or had too few electrodes, etc.) and the type of stimulus they were able to transmit was not very sophisticated.
This is why scientists working on the project began to think differently. “We went from a version based on what we knew in theory about the transmission of impulses,” continues Micera, “to a truly customised map of each patient’s injuries. In this way we knew exactly what had been lost and, as a result, how to act on the spinal cord itself, in the epidural region at the roots of the nerves”.
Highly detailed images
What Micera has just summarised is the core of years of work, during which a method of functional MRI and CT scans was developed specifically for the spinal cord. This was generally very difficult to ‘photograph’ through imaging, given the complexity of the tissue and its functions.
Therefore, after combining thousands of images from autopsies and healthy, undamaged nerves, researchers focussed on creating real maps and forecasting models of the effects of specific stimulation on the areas they thought optimal.
As Micera explains, “Calculations and simulations based on the maps showed us not only where to act, but also the type of stimulation and its intensity. This enabled us to create customised electrodes, which the team of neurosurgeons lead by Jocelyne Bloch then inserted epidurally, leading to the results we saw in the first three patients and that we hope to see in others”.
With this technique, once the implant has been inserted into the spinal cord, within just one day (a result never seen before) the patient begins to perceive the feeling of movement. On average, within a month they learn to move independently, i.e. to control how the electrodes operate via a tablet, which then controls an external device attached to the waist. This in turn interacts with a chip implanted in the abdomen, which governs the activity of the electrodes.
Finer adjustments are needed
Of course, while the programme continues, there’s a lot that still needs to be understood. For example, it is hoped that a smartphone can be used instead of a tablet, preferably with voice commands to give even more autonomy. But, above all, concludes Micera, “We want to better understand which patients are destined to recover the functions they have lost in the most satisfactory way (establishing time limits for injuries, which are difficult to regain after a certain number of months, and anatomical limits) and which, on the contrary, will not be able to benefit much from this approach.
We also want to continue refining the map development and the design of customised electronic devices as a result”.
In the meantime the U.S. Food and Drug Administration has authorised the launch of a multi-centre study to look at more cases and transfer European knowledge to colleagues overseas studying the subject, with the aim of increasing the network and the maps.
Studies are also continuing on animals in the laboratory, with direct brain implants for the same purpose.
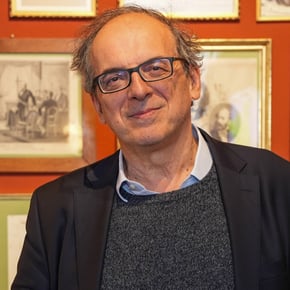